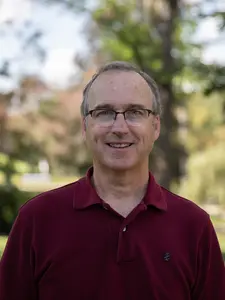
Research Statement
In my research, I use intersecting beams of laser light to slow the motion of atoms in a gas, reaching a temperature close to absolute zero, about 0.00002 Kelvin. The intersecting beams form what is called an optical lattice. We study how the forces from the lattice not only slow the atoms but can collect them into islands or rows depending on the polarization of the light.
We need to adjust our laser precisely to produce the correct wavelength of light, which has led us into the study of the physics of lasers themselves. Students have built a precision spectrometer to do this. We are learning how different pieces of the laser system help control the laser wavelength. In some cases it behaves predictably, in others it changes unpredictably, even chaotically.